Redox reactions are defined by changes in reactant oxidation numbers, and those most relevant to electrochemistry involve actual transfer of electrons. Aqueous phase redox processes often involve water or its characteristic ions, H+ and OH−, as reactants in addition to the oxidant and reductant, and equations representing these reactions can be challenging to balance. The half-reaction method is a systematic approach to balancing such equations that involves separate treatment of the oxidation and reduction half-reactions. Galvanic cells are devices in which a spontaneous redox reaction occurs indirectly, with the oxidant and reductant redox couples contained in separate half-cells. Electrons are transferred from the reductant (in the anode half-cell) to the oxidant (in the cathode half-cell) through an external circuit, and inert solution phase ions are transferred between half-cells, through a salt bridge, to maintain charge neutrality.
35.1 Review of Redox Chemistry
By the end of this section, you will be able to:
- Describe defining traits of redox chemistry
- Identify the oxidant and reductant of a redox reaction
- Balance chemical equations for redox reactions using the half-reaction method
Since reactions involving electron transfer are essential to the topic of electrochemistry, a brief review of redox chemistry is provided here that summarizes and extends the content of an earlier text chapter (see chapter on reaction stoichiometry). Readers wishing additional review are referred to the text chapter on reaction stoichiometry.
35.1.1 Oxidation Numbers
By definition, a redox reaction is one that entails changes in oxidation number (or oxidation state) for one or more of the elements involved. The oxidation number of an element in a compound is essentially an assessment of how the electronic environment of its atoms is different in comparison to atoms of the pure element. By this description, the oxidation number of an atom in an element is equal to zero. For an atom in a compound, the oxidation number is equal to the charge the atom would have in the compound if the compound were ionic. Consequential to these rules, the sum of oxidation numbers for all atoms in a molecule is equal to the charge on the molecule. To illustrate this formalism, examples from the two compound classes, ionic and covalent, will be considered.
Simple ionic compounds present the simplest examples to illustrate this formalism, since by definition the elements’ oxidation numbers are numerically equivalent to ionic charges. Sodium chloride, NaCl, is comprised of Na+ cations and Cl− anions, and so oxidation numbers for sodium and chlorine are, +1 and −1, respectively. Calcium fluoride, CaF2, is comprised of Ca2+ cations and F− anions, and so oxidation numbers for calcium and fluorine are, +2 and −1, respectively.
Covalent compounds require a more challenging use of the formalism. Water is a covalent compound whose molecules consist of two H atoms bonded separately to a central O atom via polar covalent O−H bonds. The shared electrons comprising an O−H bond are more strongly attracted to the more electronegative O atom, and so it acquires a partial negative charge in the water molecule (relative to an O atom in elemental oxygen). Consequently, H atoms in a water molecule exhibit partial positive charges compared to H atoms in elemental hydrogen. The sum of the partial negative and partial positive charges for each water molecule is zero, and the water molecule is neutral.
Imagine that the polarization of shared electrons within the O−H bonds of water were 100% complete—the result would be transfer of electrons from H to O, and water would be an ionic compound comprised of O2− anions and H+ cations. And so, the oxidations numbers for oxygen and hydrogen in water are −2 and +1, respectively. Applying this same logic to carbon tetrachloride, CCl4, yields oxidation numbers of +4 for carbon and −1 for chlorine. In the nitrate ion, , the oxidation number for nitrogen is +5 and that for oxygen is −2, summing to equal the 1− charge on the molecule:
35.1.2 Balancing Redox Equations
The unbalanced equation below describes the decomposition of molten sodium chloride:
This reaction satisfies the criterion for redox classification, since the oxidation number for Na is decreased from +1 to 0 (it undergoes reduction) and that for Cl is increased from −1 to 0 (it undergoes oxidation). The equation in this case is easily balanced by inspection, requiring stoichiometric coefficients of 2 for the NaCl and Na:
Redox reactions that take place in aqueous solutions are commonly encountered in electrochemistry, and many involve water or its characteristic ions, H+(aq) and OH−(aq), as reactants or products. In these cases, equations representing the redox reaction can be very challenging to balance by inspection, and the use of a systematic approach called the half-reaction method is helpful. This approach involves the following steps:
- Write skeletal equations for the oxidation and reduction half-reactions.
- Balance each half-reaction for all elements except H and O.
- Balance each half-reaction for O by adding H2O.
- Balance each half-reaction for H by adding H+.
- Balance each half-reaction for charge by adding electrons.
- If necessary, multiply one or both half-reactions so that the number of electrons consumed in one is equal to the number produced in the other.
- Add the two half-reactions and simplify.
- If the reaction takes place in a basic medium, add OH− ions the equation obtained in step 7 to neutralize the H+ ions (add in equal numbers to both sides of the equation) and simplify.
The examples below demonstrate the application of this method to balancing equations for aqueous redox reactions.
Balancing Equations for Redox Reactions in Acidic Solutions
Write the balanced equation representing reaction between solid copper and nitric acid to yield aqueous copper(II) ions and nitrogen monoxide gas.
Solution
Following the steps of the half-reaction method:
- Write skeletal equations for the oxidation and reduction half-reactions.
- Balance each half-reaction for all elements except H and O.
- Balance each half-reaction for O by adding H2O.
- Balance each half-reaction for H by adding H+.
- Balance each half-reaction for charge by adding electrons.
- If necessary, multiply one or both half-reactions so that the number of electrons consumed in one is equal to the number produced in the other.
- Add the two half-reactions and simplify.
- If the reaction takes place in a basic medium, add OH− ions the equation obtained in step 7 to neutralize the H+ ions (add in equal numbers to both sides of the equation) and simplify.
This step not necessary since the solution is stipulated to be acidic.
The balanced equation for the reaction in an acidic solution is then
Check Your Learning
The reaction above results when using relatively diluted nitric acid. If concentrated nitric acid is used, nitrogen dioxide is produced instead of nitrogen monoxide. Write a balanced equation for this reaction.
Balancing Equations for Redox Reactions in Basic Solutions
Write the balanced equation representing reaction between aqueous permanganate ion,
, and solid chromium(III) hydroxide, Cr(OH)
3, to yield solid manganese(IV) oxide, MnO
2, and aqueous chromate ion,
The reaction takes place in a basic solution.
Solution
Following the steps of the half-reaction method:
- Write skeletal equations for the oxidation and reduction half-reactions.
- Balance each half-reaction for all elements except H and O.
- Balance each half-reaction for O by adding H2O.
- Balance each half-reaction for H by adding H+.
- Balance each half-reaction for charge by adding electrons.
- If necessary, multiply one or both half-reactions so that the number of electrons consumed in one is equal to the number produced in the other.
This step is not necessary since the number of electrons is already in balance. - Add the two half-reactions and simplify.
- If the reaction takes place in a basic medium, add OH− ions the equation obtained in step 7 to neutralize the H+ ions (add in equal numbers to both sides of the equation) and simplify.
Check Your Learning
Aqueous permanganate ion may also be reduced using aqueous bromide ion, Br
−, the products of this reaction being solid manganese(IV) oxide and aqueous bromate ion, BrO
3−. Write the balanced equation for this reaction occurring in a basic medium.
35.2 Galvanic Cells
By the end of this section, you will be able to:
- Describe the function of a galvanic cell and its components
- Use cell notation to symbolize the composition and construction of galvanic cells
As demonstration of spontaneous chemical change, Figure 35.1 shows the result of immersing a coiled wire of copper into an aqueous solution of silver nitrate. A gradual but visually impressive change spontaneously occurs as the initially colorless solution becomes increasingly blue, and the initially smooth copper wire becomes covered with a porous gray solid.
Figure 35.1
A copper wire and an aqueous solution of silver nitrate (left) are brought into contact (center) and a spontaneous transfer of electrons occurs, creating blue Cu2+(aq) and gray Ag(s) (right).

These observations are consistent with (i) the oxidation of elemental copper to yield copper(II) ions, Cu2+(aq), which impart a blue color to the solution, and (ii) the reduction of silver(I) ions to yield elemental silver, which deposits as a fluffy solid on the copper wire surface. And so, the direct transfer of electrons from the copper wire to the aqueous silver ions is spontaneous under the employed conditions. A summary of this redox system is provided by these equations:
Consider the construction of a device that contains all the reactants and products of a redox system like the one here, but prevents physical contact between the reactants. Direct transfer of electrons is, therefore, prevented; transfer, instead, takes place indirectly through an external circuit that contacts the separated reactants. Devices of this sort are generally referred to as electrochemical cells, and those in which a spontaneous redox reaction takes place are called galvanic cells (or voltaic cells).
A galvanic cell based on the spontaneous reaction between copper and silver(I) is depicted in Figure 35.2. The cell is comprised of two half-cells, each containing the redox conjugate pair (“couple”) of a single reactant. The half-cell shown at the left contains the Cu(0)/Cu(II) couple in the form of a solid copper foil and an aqueous solution of copper nitrate. The right half-cell contains the Ag(I)/Ag(0) couple as solid silver foil and an aqueous silver nitrate solution. An external circuit is connected to each half-cell at its solid foil, meaning the Cu and Ag foil each function as an electrode. By definition, the anode of an electrochemical cell is the electrode at which oxidation occurs (in this case, the Cu foil) and the cathode is the electrode where reduction occurs (the Ag foil). The redox reactions in a galvanic cell occur only at the interface between each half-cell’s reaction mixture and its electrode. To keep the reactants separate while maintaining charge-balance, the two half-cell solutions are connected by a tube filled with inert electrolyte solution called a salt bridge. The spontaneous reaction in this cell produces Cu2+ cations in the anode half-cell and consumes Ag+ ions in the cathode half-cell, resulting in a compensatory flow of inert ions from the salt bridge that maintains charge balance. Increasing concentrations of Cu2+ in the anode half-cell are balanced by an influx of NO3− from the salt bridge, while a flow of Na+ into the cathode half-cell compensates for the decreasing Ag+ concentration.
Figure 35.2
A galvanic cell based on the spontaneous reaction between copper and silver(I) ions.
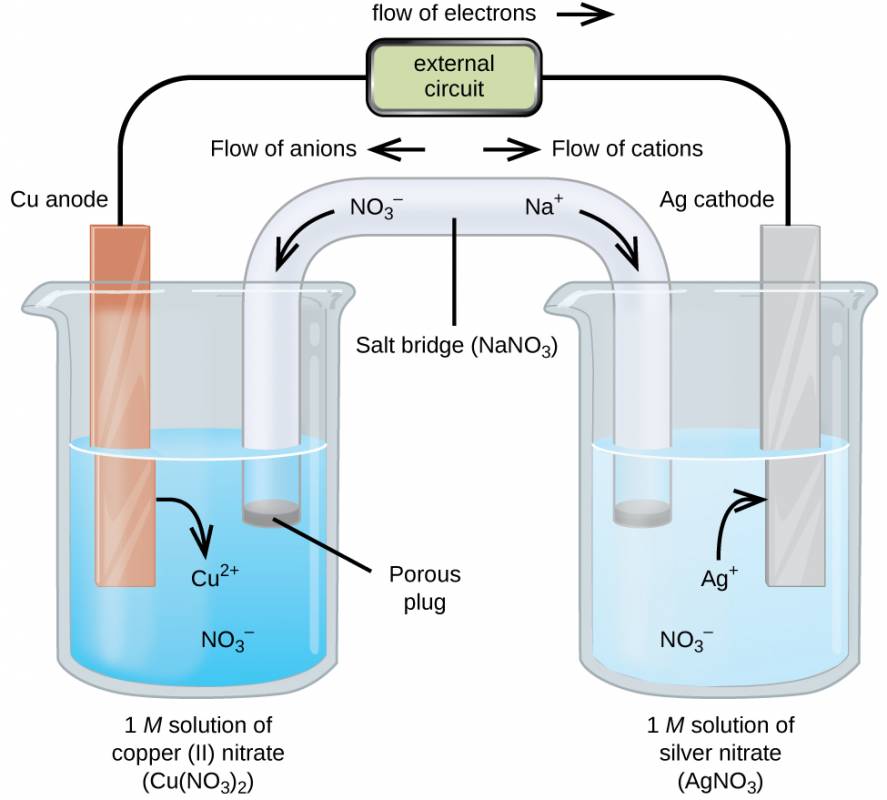
Cell Notation
Abbreviated symbolism is commonly used to represent a galvanic cell by providing essential information on its composition and structure. These symbolic representations are called cell notations or cell schematics, and they are written following a few guidelines:
- The relevant components of each half-cell are represented by their chemical formulas or element symbols
- All interfaces between component phases are represented by vertical parallel lines; if two or more components are present in the same phase, their formulas are separated by commas
- By convention, the schematic begins with the anode and proceeds left-to-right identifying phases and interfaces encountered within the cell, ending with the cathode
A verbal description of the cell as viewed from anode-to-cathode is often a useful first-step in writing its schematic. For example, the galvanic cell shown in Figure 35.2 consists of a solid copper anode immersed in an aqueous solution of copper(II) nitrate that is connected via a salt bridge to an aqueous silver(I) nitrate solution, immersed in which is a solid silver cathode. Converting this statement to symbolism following the above guidelines results in the cell schematic:
Consider a different galvanic cell (see Figure 35.3) based on the spontaneous reaction between solid magnesium and aqueous iron(III) ions:
In this cell, a solid magnesium anode is immersed in an aqueous solution of magnesium chloride that is connected via a salt bridge to an aqueous solution containing a mixture of iron(III) chloride and iron(II) chloride, immersed in which is a platinum cathode. The cell schematic is then written as
Notice the cathode half-cell is different from the others considered thus far in that its electrode is comprised of a substance (Pt) that is neither a reactant nor a product of the cell reaction. This is required when neither member of the half-cell’s redox couple can reasonably function as an electrode, which must be electrically conductive and in a phase separate from the half-cell solution. In this case, both members of the redox couple are solute species, and so Pt is used as an inert electrode that can simply provide or accept electrons to redox species in solution. Electrodes constructed from a member of the redox couple, such as the Mg anode in this cell, are called active electrodes.
Figure 35.3
A galvanic cell based on the spontaneous reaction between magnesium and iron(III) ions.
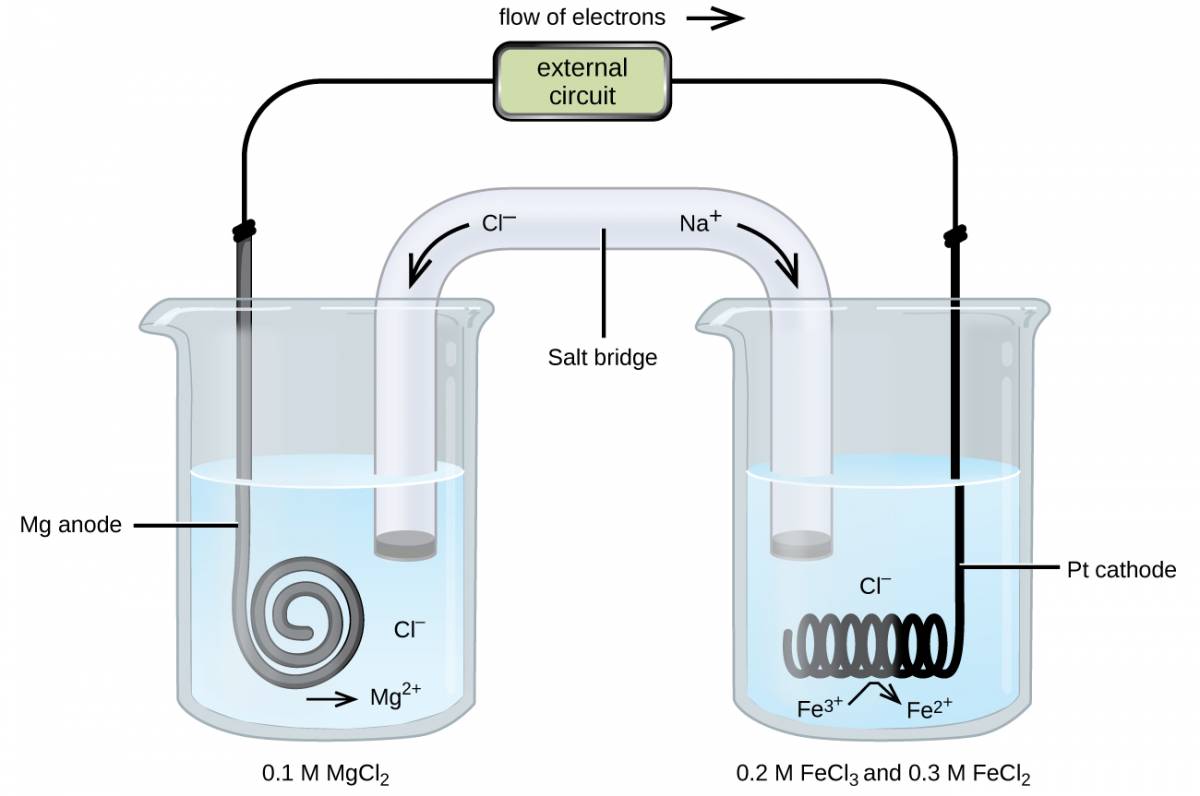
Writing Galvanic Cell Schematics
A galvanic cell is fabricated by connecting two half-cells with a salt bridge, one in which a chromium wire is immersed in a 1 M CrCl
3 solution and another in which a copper wire is immersed in 1 M CuCl
2. Assuming the chromium wire functions as an anode, write the schematic for this cell along with equations for the anode half-reaction, the cathode half-reaction, and the overall cell reaction.
Solution
Since the chromium wire is stipulated to be the anode, the schematic begins with it and proceeds left-to-right, symbolizing the other cell components until ending with the copper wire cathode:
The half-reactions for this cell are
Multiplying to make the number of electrons lost by Cr and gained by Cu2+ equal yields
Adding the half-reaction equations and simplifying yields an equation for the cell reaction:
Check Your Learning
Omitting solute concentrations and spectator ion identities, write the schematic for a galvanic cell whose net cell reaction is shown below.